Pascale Kleinfinger1,* , Laurence Lohmann1, Armelle Luscan1, Detlef Trost1, Laurent Bidat2, Véronique Debarge3, Vanina Castaigne4, Marie-Victoire Senat5,6, Marie-Pierre Brechard7, Lucie Guilbaud8, Gwenaël Le Guyader9, Véronique Satre10,11, Hélène Laurichesse Delmas12, Hakima Lallaoui13, Marie-Christine Manca-Pellissier14, Aicha Boughalem1, ylene Valduga1, Farah Hodeib1, Alexandra Benachi5,15 and Jean Marc Costa1
- ラボラトリア CERBA, 7/11 Rue de l’Equerre, 95310 Saint-Ouen-l’Aumone, France;
- llohmann@lab-cerba.com (L.L.); armelle.luscan@lab-cerba.com (A.L.); dtrost@lab-cerba.com (D.T.); Aicha.Boughalem@lab-cerba.com (A.B.); Mylene.Valduga@lab-cerba.com (M.V.); Farah.Hodeib@lab-cerba.com (F.H.); jmcosta@lab-cerba.com (J.M.C.)
- 婦人科オブステトリック、フランス・デュボス中央病院、フランス・デル・デル・オブ・フランス、95300ポントイズ、フランス・lbidat@wanadoo.fr 婦人科・オブステトリック、CHU リレ、2アブオスカーラブレット、59000リレ、フランス、veronique.debarge@chru-lille.fr フランス・クレテイル市内(第四十条、第九百四十〇条)及びVanina.Castaigne@chicreteil.fr パリ大学医学部、63 Rue Gabriel Peri, 94270 Le Kremlin-Bicetre, France; marie-victoire.senat@aphp.fr (M.-V.S.); alexandra.benachi@aphp.fr (A.B.) 婦人科-Obstetrique, Hopital Bicetre, 78 Rue du General Leclerc, 94270 Le Kremlin-Bicetre, France
- 細胞遺伝学、聖ジョセフ病院、ブールバード・ド・ルーヴァン、13008 マルセイユ、フランス、26歳、mbrechard@hopital-saint-joseph.fr
- メデシン胎児サービス、ホータルマンドトルソー、APHPソルボンヌ大学トロソー、フランス、パリ、75012パリ、ドクターアーノルドネットター、26街、lucie.guilbaud@gmail.com
- ジェネティックメディケル、CHU de Poitiers, 2 rue de la Miletrie, CEDEX, CS 90577, 86021 Poitiers, France; gwenael.leguyader@chu-poitiers.fr
- 遺伝的染色体、CHU Grenoble Alpes, Avenue Maquis du Gresivaudan, 38700 La Tronche, France; vsatre@chu-grenoble.fr
- INSERM U1209, CNRS UMR 5309, Institute for Advanced Biosciences, Team Genetics Epigenetics and Therapies of Infertility, Univ. フランス、38700ラ・トロンシュ、グレノブル・アルペス、アベニュー・マキス・ド・グレシバウダン、グレノブル・アルペス
- 産婦人科・産婦人科医、CHU Clermont Ferrand, and Place Lucie et Raymond Aubrac, 63003 Clermont Ferrand, France; helaurichesse@chu-clermontferrand.fr
- Cylab, 6 Rue des sports BP 60348, CEDEX 1, 17001 La Rochelle, France; hlallaoui@cylab.fr
- 出生前診断センター、Timone病院、264 Rue Saint-Pierre, 13005 Marseille, France; manca-pellissier@ap-hm.fr
- 婦人科-Obstetrique, Hopital Antoine Beclere, AP-HP, 157 Rue de la Porte de Trivaux, 92140 Clamart, France
* Correspondence: PKleinfnger@lab-cerba.com; Tel.: +33-1-34-40-20-78
Received: 8 July 2020; Accepted: 28 July 2020; Published: 1 August 2020
summary:
Atypical fetal chromosomal abnormalities are more frequent than previously recognized and are possible during rectal fetal development. We proposed a screening strategy for genome-wide noninvasive prenatal testing (NIPT) to detect these atypical chromosomal abnormalities (ACA). Two sample cohorts were tested. Analytical performance was measured using cohort A, consisting of 192 biobanked plasma samples with ACA, 42 with ACA and 150 without ACA. The rate of additional invasive diagnostic procedures was determined using cohort B, consisting of 3097 pregnant women referred for routine NIPT. Of the 192 samples in cohort A, there were four initial test failures and six discordant calls, resulting in an overall sensitivity of 88.1% (37/42; CI 75.00 to 94.81) and specificity of 99.3% (145/146; CI 96.22 to 99.88). In cohort B, there were 90 frst-pass failures (2.9%). When restricted to significant unbalanced chromosomal abnormalities and trisomies 8, 9, 12, 14, 15, 16, and 22, the rates of abnormal positive results were 1.2% (36/3007) and 0.57% (17/3007). These results indicate that genome-wide NIPT is feasible for screening for ACA with acceptable sensitivity and a modest increase in invasive testing, especially by limiting screening to structural abnormalities and the most clinically meaningful trisomies, with increased risk after maternal serum screening.
keyword:
Noninvasive prenatal testing; Genome-wide screening strategies; Atypical chromosomal abnormalities; Rare autosomal aneuploidies; Structural imbalances; Deletions; Duplications; Sensitivity; Specificity; Positive predictive value; VeriSeq™ NIPT Solution v2
1. Introduction
Several tests are available to screen for chromosomal abnormalities. The most common is traditional maternal serum screening (MSS) in the first or second trimester, which typically screens for common fetal aneuploid anomalies (trisomies 21, 18, and 13) and provides a risk score to determine whether further testing is necessary. The risk of atypical chromosomal anomalies (ACAs), namely rare autosomal aneuploid anomalies (RAAs) and structurally unbalanced anomalies (SUAs), has been shown to increase with the MSS risk score [1-3]. Ultrasound examination, including transurethral translucency measured between 11 and 13 weeks of gestation, can detect some chromosomal abnormalities. More recently, noninvasive prenatal testing (NIPT) has become available. NIPT can be performed at any stage of pregnancy, generally from 10 weeks gestation onwards, to ensure sufficient fetal fraction (FF) in the maternal plasma sample [4]. NIPT is typically used to screen for common trisomies. Genome-wide screening for ACA is also possible with NIPT assays using whole genome sequencing (WGS) and analysis.[5,6] Invasive procedures such as chorionic villus sampling (CVS) or amniocentesis to perform fetal karyotype or microarray are considered diagnostic but carry a small risk of miscarriage[7,8] and may carry a risk of premature rupture of membranes and chorioamnionitis[9,10].
Clinical studies have shown improved performance of NIPT over traditional MSS approaches [11]. Several different technologies are commonly used for NIPT assays, mainly massively parallel WGS [12-14], targeted microarray hybridization [15], and SNP-based sequencing [16,17]. Massively parallel sequencing technologies use single-end or paired-end sequencing, where paired-end sequencing can determine both fragment size and location. NIPT performance can vary by method, and a recent meta-analysis [20] reported that NIPT failure rates range from 0.1 to 6.3% depending on the method [21-23].
In recent years, the options in the testing menu have expanded to include sex chromosome aneuploidies [24,25], selective microdeletions/duplications [26,27], and ACAs [5,6,28]. Although ACAs are rarer, NIPT studies have shown screen positivity rates of 0.1% for SUAs and 0.34% for RAAs [29]. In a 2017 study by Fiorentino et al. [5], genome-wide cell-free DNA analysis in a large cohort of pregnant women identified clinically relevant imbalances that could not be detected by conventional NIPT, while maintaining high specificity. A study by Pescia et al. [30] also revealed that the use of genome-wide NIPT screening has led to an increase in the detection of fetal anomalies, and that rare autosomal trisomies and CNVs identified by this screening are important causes of fetal pathology. In France, these ACAs account for 12.25% of all prenatal unbalanced chromosomal abnormalities and 2.5% of all prenatal karyotypes [31]. These ACAs have been shown to be associated with poor outcomes, including miscarriage, intrauterine fetal death, intellectual developmental disability, and malformation syndromes [29,32-35].
In France, NIPT is only reimbursed for trisomy 21 screening. NIPT is performed as a second-tier screening following MSS with an MSS risk score between 1/51 and 1/1000, and as a first-tier screening for women with multiple pregnancies, a history of a previous pregnancy affected by trisomy 21, or in whom either parent is known to be a carrier of a Robertsonian translocation involving chromosome 21. Diagnostic testing is recommended if ultrasound is abnormal or if the MSS risk score is greater than 1/50.
The objectives of this study were (1) to determine the performance of genome-wide cell-free DNA analysis with the VeriSeq™ NIPT Solution v2 assay for the detection of ACA and (2) to determine a strategy for genome-wide NIPT screening for ACA. Our study found that this genome-wide NIPT assay showed high accuracy in detecting rare autosomal aneuploidies and segmental aneuploidies of 7 Mb or more, minimizing the increase in additional confirmatory invasive testing. We also suggest a strategy to limit genome-wide screening to the most important chromosomal trisomies and SUA and to offer it to high-risk women after maternal serum screening.
2. Experimental Section
The study included two study cohorts. Cohort A consisted of a biobank of plasma samples collected to determine the sensitivity and specificity of the genome-wide NIPT assay. Abnormal samples were collected between 2014 and 2019 from patients who had undergone invasive surgery and whose karyotype or microarray (CMA) revealed abnormalities other than common trisomies (trisomies 21, 18, and 13) or sex chromosome aneuploidies (Supplementary Table S1). All patients with karyotype/microarray abnormalities were offered to participate in the study without selection based on the type of abnormality, indication for invasive procedure, or gestational age. This group represents an unselected group among the chromosomal abnormalities encountered in our cytogenetic laboratory. Control NIPT samples in cohort A consisted of the first 148 unselected samples collected from April to July 2019 with karyotype/microarray results without abnormalities other than common trisomies or sex chromosome aneuploidies. NIPT samples were collected at the same time as the invasive surgery. Because amniotic fluid karyotype was normal and mosaicism localized to the placenta (chorioblasts) was diagnosed, two supplemental NIPT samples were taken after the karyotype results.
Cohort B, further referred to as the “referred population”, was collected with the aim of determining the proportion likely to undergo additional invasive diagnostic procedures after the use of genome-wide NIPT. It consists of unselected samples from patients undergoing routine NIPT for common trisomies as part of their clinical workup. Genome-wide NIPT analysis was subsequently performed for this study. As patients consented to a non-interventional study only, no genome-wide analysis results were reported and patients were not contacted to obtain further follow-up information regarding fetal karyotype and clinical outcome. These samples were collected between March and August 2019. All samples in both cohorts were from singleton pregnancies.
For cohort A, karyotyping was performed using standard techniques: in situ culture (Amniomax®) or trypsin culture (Amniomedium 2® for amniotic fluid and Amniomax® for trophoblastic cultures), hypotonic shock (Hanks® and MgCl2), fixation with acetic acid/methanol, and RHG banding. At least 15 metaphases of the two cultures were analyzed. Microarrays were performed using a genome-wide array Cytoscan® 750K (SNP Affymetrix, 750K markers) according to the Affymetrix protocol.
All samples were sequenced using the CE-IVD marked VeriSeq™ NIPT Solution v2 assay (Illumina, Inc.). This assay uses a PCR-free paired-end WGS approach to detect genome-wide abnormalities. Briefly, plasma samples from both the biobank and the referral population were frozen, followed by thawing the plasma and a cell-free (cf) DNA extraction step. Purified cfDNA fragments underwent automated library preparation using the VeriSeq™ NIPT Microlab STAR system, followed by quantification and library pooling. Pooled libraries were sequenced on a NextSeq 550 Dx sequencer (Illumina, Inc.). Biobanked samples were tested on the same flow cells used for the referred population samples, with a maximum of four biobank samples per flow cell. Bioinformatics analysis was performed using the VeriSeq™ NIPT Solution v2 system server, and samples were randomly selected for ≥7 RAAs or SUAs. For the presence of Mb, results were classified as abnormality detected or abnormality not detected. The assay software uses a dynamic threshold metric known as the individualized fetal annullity confidence test (iFACT), which takes into account both fetal fraction and coverage information to determine whether a call can be made, allowing for accurate calls at low fetal fractions.
Comparisons of fetal fraction and gestational age between different patient populations were performed using t-tests; a p-value of <0.05 was considered significant. Binomial 95% confidence intervals (CIs) were calculated for performance estimates.
The study was approved by the Committee for the Protection of Human Beings (N◦ PP 14-007). Women gave written consent that their samples may be used in research and that the results of the study would not be communicated to them.
3.Results
Cohort A, the biobank sample, consisted of a total of 192 samples (Figure 1). In 42 pregnancies, RAA or SUA ≥ 7 Mb were detected by invasive testing (Supplementary Table S1): 4 RAA (3 trisomy 16 (type III, i.e., detected in both cytotrophic cells and mesenchyme) and 1 non-mosaic trisomy 22 confined to the placenta), 32 SUA (duplication, deletion, translocation-inducing chromosomes), 5 markers (3 i(12)(p10), 1 i(18)(p10), and 1 pathologically derived chromosome 15), and 1 chromoanagenesis (multiple complex rearrangements at one or more chromosomal loci) [36]. Thirteen patients were diagnosed by CMA and karyotype, two by CMA alone, and 27 by karyotype alone. Nineteen patients were diagnosed based on amniotic fluid (AF), 18 on CVS, and five had two or more histologies available for diagnosis. The other 148 specimens were classified as unaffected (i.e., RAA or SUA ≥ 7 Mb), 39 had microarray, and 109 had karyotype. The remaining two specimens had abnormal cytoblasts and normal fetoplacental discordance. The mean gestational age was 17.7 weeks (range 11.0-36.3 weeks).
Figure 1. Overview of biobank samples. Overview of the study population, failed samples, and final NIPT cohort. †Two cases had placental discordance between abnormal cytoblasts and normal fetuses. The first case was mosaic 46,XY,del(5)(p15)(7)/46,XY(12) by direct cytoblast karyotype and mosaic 46,XY by long-term culture and amniotic fluid culture. The second case was 47,XY,+der(13)t(11;13)(q12;q34)/46,XY,t(11;13) by direct cytoblast karyotype and 46,XY,t(11;13)(q12;q34) by long-term culture and amniotic fluid culture.
The failure rate was 2.08%, as samples could only be performed once due to sample constraints. All four failed samples were from pregnancies with normal fetal cytogenetic results. Of the 188 cases, there were 150 low-risk and 38 high-risk NIPT calls. The mean FF was 11.76%. There was no statistical difference between normal and abnormal samples in the biobank population (p = 0.52).
There was one false positive call and five false negative calls. The sensitivity for detecting RAAs and SUAs ≥ 7 Mb was 88.1% (37/42; CI 75.00-94.81) and specificity 99.32% (145/146; CI 96.22-99.88). One false positive call was found to be due to focal placental mosaicism type 1 (CPM1, i.e., cytotrophic cells only). This sample was reported by NIPT as dup(11)(p11.12q25); + 13. The karyotype of the direct analysis of the cytotrophic cells was 47,XY,+der(13)t(11;13)(q12;q34),t(11;13), whereas the karyotype of the mesenchymal long-term culture was 46,XY,t(11;13)(q12;q34).
An overview of the five false-negative results is shown in Table 1; the FF for these samples ranged from 3 to 15%. The rearrangement in sample 1 was a deletion of part of band 4p16.3, the size of which was not examined by the array. It was visible on the 550 RHG banding karyotype (resolution 5-10 Mb), but the size of the deletion could be between 5 and 7 Mb, which is one possible explanation for the false-negative NIPT result, since it is below the minimum size threshold for detection with this NIPT assay. In sample 2, the false-negative result is due to the presence of a suspected mosaicism due to isochromosome 12p (OMIM 601803), as is usually seen in Pallister-Killian syndrome. For sample 3, chromosomal synthesis is the most likely reason for the false-negative result. We could not provide a biological explanation for the false-negative result in sample 4, but the low FF may explain the discordance. For sample 5, a combination of low FF and possible mosaicism (chromosome described as postzygotic) may explain the false-negative result.
Sample | Fetus proportion | Karyotype | Size (Mb) | Comments |
---|---|---|---|---|
1 | 10% | 46,XX,del(4)(p16.3).ish del(4)(WHS-,D4S3359-) | 5-8 based on karyotype | Possible size <7 Mb |
2 | 15% | arr[GRCh37] 12p13.33q11(173786_37876500)x3 | 37.7 | Suspected mosaic phenomenon |
3 | 9% | 46,XX,der(8)?add(8)(p?)?dup(8)(q22q23)dn.ish der(8)(qter->?::?->qter)(D8S504, VIJyRM2053+,wcp8+,VIJyRM2053+).arr[GRCh38] 8p23.3p23.1(158048_6935930)x1,8p23.1p11.23(12585435_ 38267493)x3, 8p11.22(38314367_39246760)x3, 8p11.22(39247087_39386852)x1,8p11.22(39389765_40264413)x3, 8q22.3q23.2(104688373_111952230)x3, 8q24.3(144972747_146295771)x3 | Total deletion=6.9 Total duplicates=36.2 |
Chromona synthesis |
4 | 3% | 46,XX,add(4)(qter).ish add(4)(wcp4-).arr[GRCh37] 5q31.2q35.3(138522878_180715096)x3 | 41.2 | |
5 | 4% | 46,XY,i(18)(q10) |
In samples with microarray results (15 rearrangements), a good correlation was observed between the segment sizes of partial unbalanced rearrangements detected by NIPT and those detected by microarray, with one exception (sample 5 in Table 2). NIPT called this sample trisomy 11, whereas karyotype and array reported a derivative chromosome 11 from the translocation t(11;12), with a partial terminal deletion of chromosome 11 of 2.7 Mb and a partial terminal duplication of trisomy 12 of 11.7 Mb.
Sample | NIPT | Arrangement |
---|---|---|
1 | 18.2 | 12.8 |
2 | 9.7 | 9.8 |
3 | 11.5 | 11.1 |
4 | 28.8 | 29.9 |
5 | Trisomy 11 | 2.7 |
6 | 8.1 | 6.7 |
7 | 10.7 | 11.5 |
8 | 11.3 | 17.4 |
9 | 26 | 26.6 |
10 | 17.2 | 7.9 |
11 | 18.3 | 18.3 |
12 | 9 | 8.8 |
13 | 12.5 | 12.5 |
14 | 13.7 | 11.8 |
15 | 60.1 | 59.9 |
CNV, copy number variation; NIPT, non-invasive prenatal test. |
Analysis of 41 deletion/duplication segments ≥ 7 Mb in 35 fetuses with partially unbalanced rearrangements suggests that detection rates are comparable for deletions and duplications. In contrast, the sensitivity may be higher for interstitial translocations compared with terminal translocations (Table 3), but a larger sample size is needed to confirm this trend.
Types of dislocation | Karyotype n inspection | Detected by NIPT, n | Detection rate, % (95% confidence interval) |
---|---|---|---|
Delete | 13 | 11 | 84.6 (54.6-98.1) |
Copy | 28 | 24 | 85.7 (67.3-96.0) |
Interstitial | 5 | 5 | 100 (47.8-100) |
Terminal | 36 | 28 | 77.8 (60.9-89.9) |
CI, confidence interval |
We also wanted to determine a screening strategy to select patients at highest risk of adverse outcomes based on the MSS risk score. Lindquist et al. [3] determined prevalence rates based on the MSS risk score. Using these prevalence values, we calculated the positive predictive values (PPVs) of different MSS risk scores using our study specifications of 99.32% and sensitivity of 88.1% (Table 4). Theoretical PPVs ranged from 11% to 64% for the general population and for the group with an MSS risk score >1/300.
Measurement | General population | MSS score 1/51-1/1000 |
MSS score >1/1000 |
MSS score 1/51-1/300 |
MSS score >1/300 |
---|---|---|---|---|---|
Prevalence rate1 | 0.10% | 0.37% | 0.61% | 1.01% | 1.40% |
PPV | 11% | 32% | 44% | 56% | 64% |
1Values are based on the study of Lindquist et al. [3]. PPV, positive predictive value; ACA, atypical chromosomal abnormality; MSS, maternal serum screening. |
Cohort B, the referral population cohort (Figure 2), consisted of 3097 samples from women who underwent routine NIPT for common aneuploidies, 88% of these performed for MSS risk ≥ 1/1000 (Table 5). The mean gestational age was 16.8 weeks (10.1 vs. 37.0 weeks). The referral population (cohort B) had a slightly lower mean gestational age than the biobank (cohort A) samples (16.8 vs. 17.7 weeks, respectively; p = 0.003). Gestational ages in the biobank cohort ranged from 11.0 to 36.3 weeks. Comparing the fetal fractions of cohort A and cohort B (Table 6), significant differences were noted (p < 0.001).
Population type | Failed | No abnormality | Common trisomy | ACAs | Total, n(%) | |
---|---|---|---|---|---|---|
MSS≧1/1000 | 71 | 2596 | 35 | 29 | 2731(88) | |
MSS < 1/1000 | 9 | 139 | 0 | 1 | 149(5) | |
Parental Robertsonian translocation | 0 | 2 | 0 | 0 | 2 (0) | |
History of fetal trisomy | 2 | 51 | 0 | 1 | 54 (2) | |
One review | 8 | 147 | 1 | 5 | 161 (5) | |
Total | 90 | 2935 | 36 | 36 | 3097 | |
ACA, atypical chromosomal abnormality; MSS, maternal serum screening. |
Measurement | Biobank sample (cohort A) (n = 189) | Normal biobank samples from cohort A (n = 147) | Abnormalities in biobank samples from cohort A (n = 42) | Query sample (cohort B) (n = 3007) |
---|---|---|---|---|
Average | 12.27% | 12.40% | 11.76% | 11% |
Median value | 11% | 11% | 10.5% | 10% |
Range | 3-35% | 4-35% | 3-24% | 2-35% |
NIPT analysis of a sample of 3097 points (Cohort B) resulted in a first failure of 90 points (2.9%); the final failure rate is unknown as the sample did not take the second passing exam. Of the 3007 specimens for which results were received (Figure 2), 36 specimens (1.2%) had positive results for trisomies 13, 18, and 21, and 36 specimens (1.2%) had positive results for ACA. In the high-risk population (MSS risk ≥1/1000), 1.09% were ACA positive, and in the primary screening population, 3.27% were positive (Table 7). The 36 ACAs consisted of 10 SUAs, 25 RATs, and 1 case with multiple abnormalities. namely, (1) deletions, duplications, translocation derivative chromosomes, inversions or recombinants of marker chromosomes, and (2) SUAs corresponding to classic chromosomal rearrangements such as trisomies 8, 9, and 22; uniparental trisomy 14 and 15 for the risk of disomy; trisomy 16 for the risk of poor pregnancy outcome; and Trisomy 12 (Pallister Killian syndrome) for isochromosome 12p risk; only 17 samples were positive: only 0.57% in the total study group, 0.53% in the high-risk population with MSS risk ≥1/1000, and 0.65% in the population with further NIPT screening (Table 7).
Population type | Total excluding failures | Group 1+SUA | Group 2 | Group 3 | Prevalence of all ACA | Group 1+SUA prevalence |
---|---|---|---|---|---|---|
MSS≧1/1000 | 2660 | 14 | 10 | 5 | 1.09% | 0.53% |
MSS < 1/1000 | 140 | 1 | 0 | 0 | 0.71% | 0.71% |
One review | 153 | 1 | 3 | 1 | 3.27% | 0.65% |
History of fetal trisomy or parental Robertsonian translocation | 54 | 1 | 0 | 0 | 1.85% | 1.85% |
ACAs, atypical chromosomal abnormalities; MSS, maternal serum screening. Group 1 includes the following trisomies: trisomies 8, 9, and 22, because these trisomies often involve the fetus; trisomies 14 and 15, because of the risk of uniparental disomy; trisomy 16, because of the risk of multiple adverse pregnancy outcomes; and trisomy 12, because homozygous chromosome 12p causes Pallister-Killian syndrome. Group 2 includes trisomies 3 and 7, which are known to be frequently associated with trophoblast cells [37]. Group 3 includes all other RAAs (trisomies 1, 2, 4, 5, 6, 10, 11, 17, 19, and 20), including trisomies that are nonpathogenic to the fetus, such as mosaic trisomy 20, or that are commonly attached to the placenta.
4. Investigation
In this paper, we evaluated the ability of genome-wide NIPT assays to detect the presence of atypical chromosomal abnormalities. NIPT was found to be highly specific for their detection, and CPM was corroborated as a biological explanation for a single false-positive call. Genome-wide NIPT screening detected the majority of unbalanced rearrangements in the biobank population with a sensitivity of 88.1% (CI 75.00.94.81) and showed good correlation with the array for the CNV size detected. Although it was lower than the sensitivity observed for common trisomies [38], biological explanations were suspected for three of the false-negative results (large size of deletion, suspected mosaicism, and pigmented rhinopharyngeal syndrome). Due to the high specificity, the number of samples in the referral population that received positive results for the presence of ACA was small (1.2%). Failure rates in clinical practice are expected to be lower than those observed in this study due to the ability to run additional plasma samples if the first sample fails. The test (biobank) samples were slightly longer gestational age than the referral population, and as expected, the mean fetal fraction was slightly higher. However, the di.erence between the two populations was not clinically meaningful and therefore performance in the study population is likely to be similar to that observed in the general pregnant population.
Although there is a consensus on the value of screening for common fetal aneuploidies, the usefulness of screening for atypical fetal anomalies remains hotly debated [39] and remains controversial in the clinical community [40]. Even when the poor prognosis and diagnostic utility of these ACAs are considered to be associated, they are generally considered to be too rare to be part of a screening policy. In fact, the exact prevalence of these conditions in the population remains unknown and is probably underestimated, because there is no widespread availability of invasive testing in large unselected pregnant populations, nor are there any studies routinely testing all newborns for these anomalies. In a recent report from the French Institute of Cytogenetics [31], ACAs accounted for 12.25% of prenatal unbalanced chromosomal anomalies. According to Lindquist et al. [3], the overall prevalence is estimated to be 1/1000, not taking into account cases that may have been detected after birth. Moreover, it has been shown that the risk of ACA increases with the MSS risk score [1.3], making its use as a secondary test even more important.
Our study shows that genome-wide NIPT is an effective method of screening for atypical fetal anomalies, such as rare trisomies and segmental imbalanced rearrangements, and may allow the detection of more fetal anomalies without significantly increasing unnecessary invasive procedures. Two strategies may contribute to selecting the population at highest risk of adverse outcomes: (1) recommending genome-wide NIPT to pregnant women at high risk based on MSS results, as outlined in the results section above, and (2) screening for a limited number of ACAs known to be at high risk of adverse outcomes. Regarding the second strategy, although screening for ACAs with genome-wide NIPT is possible in the general pregnant population, we suggest limiting the implementation of genome-wide NIPT to pregnant women with an MSS risk score ≥ 1/1000 (theoretical PPV 32–64%). This recommendation is possible because in France, for singleton pregnancies without a history of chromosomal rearrangements, NIPT is a second-tier screening test conditional on the MSS risk score. Restricting genome-wide NIPT to pregnant women with high-risk MSS scores is also a possibility in other countries in Europe, such as the Nordic countries, Poland, Romania, Italy, and Australia [41]. Other countries may choose to use genome-wide cfDNA analysis in unselected populations. There is a large variation in how countries approach NIPT, with some recommending NIPT as a first-line option and others as part of an incidental model. Benachi et al. [42] report the results of a survey of European healthcare professionals and highlight the large differences between healthcare professionals in each country with regard to expanding NIPT options, such as screening for rare autosomal trisomies and copy number variations. It is important to note that reimbursement for NIPT at a national level would be a valuable part of prenatal screening, but is currently only possible in certain countries, such as those with national or regional reimbursement programs that fund NIPT. In other countries, patients may be required to pay for NIPT, or it may be covered by the patient’s health insurance.
For the second strategy, rare autosomal aneuploidies can be classified into three groups. Group 1 includes trisomies 8, 9, and 22, which are most frequently involved in the fetus; trisomy 14 and 15 because of the risk of monoparental discord; trisomy 16 because of the risk of multiple adverse pregnancy outcomes; and trisomy 12 because isochromosome 12p causes Pallister-Killian syndrome. Group 2 includes the trisomies known to be most frequently bound to cytotrophoblasts, namely trisomies 3 and 7 [37]. Group 3 consists of all other RAAs, including trisomies that are nonpathogenic for the fetus, such as mosaic trisomy 20, or trisomies that are commonly bound to the placenta. As screening tests must detect abnormalities of a certain prevalence, we recommend using genome-wide NIPT to screen for RAAs in group 1 only. This strategy is particularly interesting in low-risk populations (first-tier screening), since in our cohort, four of the five anomalies belonged to groups 2 and 3, even if the numbers were too small to draw definitive conclusions. Reanalysis of the cohort published in a recent study of the Dutch NIPT Consortium [28] would have revealed that only 47 women, instead of 101, would have had a positive NIPT call for RAAs, and all cases with fetal RAAs would have been diagnosed. In our study, targeting only SUA and trisomies 8, 9, 12, 14, 15, 16 and 22 resulted in a positive NIPT call rate of 0.57% (17/3007).
The sensitivity of genome-wide NIPT is not high enough to recommend it for screening fetuses with ultrasound abnormalities, especially given the fact that microdeletions/duplications are frequently involved. Therefore, we recommend that patients with fetal structural abnormalities on ultrasound undergo diagnostic invasive testing with microarray analysis.
One of the main strengths of this study was the availability of samples affected by chromosomal abnormalities other than common trisomies and that the fetal karyotype of all biobank samples was known. This allowed us to determine the true study specificity and sensitivity. We observed a high specificity of 99.32% (CI 96.22-99.88) with only one false-positive result by NIPT, determined to be due to CPM; CPM is a known biological factor that affects the clinical specificity of NIPT. The availability of a referral population of routine pregnancy samples was also a strength. Using this population and set strategy, we were able to show that the use of whole-genome NIPT does not result in a significant increase in unnecessary invasive diagnostic procedures, a typical argument against genome-wide NIPT screening. Here, the NIPT positive call rate for the presence of ACA did not exceed 1.2%, which is comparable to other studies [33]. Moreover, by selecting the most significant ACA, we were able to reduce the positive call rate to 0.57%.
One of the main study limitations was that this was a selected set of pregnancy samples and therefore may not be truly representative of the larger general pregnancy population. Other recent publications have examined genome-wide NIPT in populations of at least 10,000 patients [5,6,34,43], while some publications report results from populations of more than 50,000 patients [28,44,45]. However, the assay failure rates and FF distributions observed in our study population are consistent with those observed in the referred population, suggesting that the data are representative. Another limitation of the present study was the lack of karyotype and clinical follow-up information for the referred population. Although the purpose of the referred population was only to calculate a potential increase in invasive procedures, it may be interesting to have that information to determine the positive and negative predictive values of this genome-wide NIPT assay and to investigate whether genome-wide NIPT is cost-effective. Larger studies targeting different pregnancy complications may provide further information on which RAAs should be screened for with NIPT. Additionally, further studies are needed to determine whether genome-wide NIPT could be an effective prenatal screening option for detecting rare fetal abnormalities in multiple pregnancies.
5. Conclusion
In conclusion, this study showed that the genome-wide NIPT assay is a valid non-invasive method to screen for the presence of ACA in pregnant women. We propose to screen only the most significant chromosomal trisomies and SUA. We propose that in France, genome-wide NIPT screening be considered for all pregnant women with normal ultrasound findings after a high-risk MSS result.
Supplementary Material
http://www.mdpi.com/2077-0383/9/8/2466/s1, Table S1: Summary of the 42 abnormal samples from Cohort A is available online.
Author contributions:
Conceptualization, PK, LL. and JMC.; methodology, PK, LL, AB. (Alexandra Benachi) and JMC.; validation, PK, LL, AB. (Alexandra Benachi) and JMC.; investigation, PK, LL, AL, DT, LB, VD, VC, M.-VS, M.-PB, LG, GLG, VS, HLD, HL, M.-CM-P., AB. (Aicha Boughalem), MV, FH, AB. (Alexandra Benachi), and JMC.; resources, PK, LL, AL, DT, AB. (Aicha Boughalem), MV. and JMC.; preparation of original draft, PK, AB. (Alexandra Benachi); writing, review and editing, PK, AB. (Alexandra Benachi), and JMC.; supervision: PK, AB. (Alexandra Benachi) and JMC. ; project administration, PK and FH. All authors have read and agreed to the published manuscript.
Funding:
This study received no external funding.
Acknowledgements:
The authors would like to thank Kristine Jinnett (Ilumina, Inc.) for assistance with collecting biobank samples, as well as Nicole Joye (Hôpital Trousseau, Paris) and Elisabeth Simom (Fofation Lenval, Nice) for their assistance in preparing this manuscript.
Declaration of interest:
The authors declare that they have no identification of interest.
References
- Huang, S.;Chang, C.;Cheng, P.; Hsiao, C.; Soong, Y.; Duan, T. First-trimester combined screening is effective for detecting chromosomal unbalanced translocations at 11-12 weeks of pregnancy. Regret. Sci. 2014, 21, 594-600. [CrossRef]
- Toring, N.; Petersen, OB; Becher, N.; Vogel, I.; Uldbjerg, N. Early pregnancy screening for trisomies other than trisomies 21, 18, and 13. Prenatal. Diagn. 2015, 35, 612-619. [CrossRef]
- Lindquist, A.; Poulton, A.; Halliday, J.; Hui, L. Atypical chromosomal abnormalities after combined prenatal diagnostic testing and early-trimester screening: Implications for incident models of noninvasive prenatal testing. Ultrasound. Gynecol. 2018, 51, 487-492. [CrossRef]
- Bianchi, DW; Chiu, RWK Sequencing of circulating cell-free DNA during pregnancy. N. Engl. J. Med. 2018, 379, 464-473. [CrossRef] [PubMed]
- Fiorentino, F.;Bono, S.;Pizzuti, F.;Duca, S.;Polverari, A.;Faieta, M.;Baldi, M.;Diano, L.;Spinella, F.;Clinical utility of genome-wide noninvasive prenatal screening. Prenatal. Diagn. 2017, 37, 593-601. [CrossRef] [PubMed]
- Liang, D., Lin, Y., Qiao, F., Li, H., Wang, Y., Zhang, J., Liu, A., Ji, X., Ma, D., Jiang, T., et al. Perinatal outcomes after cell-free DNA screening in over 32,000 women: Clinical follow-up data from a single tertiary center. Diagn. 2018, 38, 755-764. [CrossRef]
- Akolekar, R.; Beta, J.; Picciarelli, G.; Ogilvie, C.; D’Antonio, F. Risks associated with miscarriage after amniocentesis and chorionic villus sampling: a systematic review and meta-analysis. Ultrasound. Gynecol. 2015, 45, 16-26. [CrossRef] [PubMed]
- Solomon, LJ; Sotiriadis, A.;Wul., CB; Odibo, A.; Akolekar, R. Risk of miscarriage after amniocentesis or chorionic villus sampling: a systematic review of the literature and an updated meta-analysis. Ultrasound. Gynecol. 2019, 54, 442-451. [CrossRef]
- Michael, T.;Natalia, S.-L.;James, M.;Jg, B.; Yuri, N.; Andreas, SW; Gregor, S. Mid-trimester premature rupture of membranes (PPROM): international recommendations on etiology, diagnosis, classification, treatment options and outcomes. J. Perinat. Med. 2018, 46, 465-488. [CrossRef]
- Cederholm, M.; Haglund, B.; Axelsson, O. Maternal complications after amniocentesis and chorionic villus sampling for prenatal karyotyping. BJOG 2003, 110, 392-399. [CrossRef]
- Norton, ME; Baer, RJ; Wapner, RJ; Kuppermann, M.; Jelli.e-Pawlowski, LL; Currier, RJ Cell-free DNA versus sequential screening for detection of fetal chromosomal abnormalities. US J Obstet Gynecol. 2016, 214, e1-e6. [CrossRef] [PubMed]
- Sehnert, AJ; Rhees, B.; Comstock, D.; de Feo, E.; Heilek, G.; Burke, J.; Rava, RP Optimal detection of fetal chromosomal abnormalities by massively parallel sequencing of cell-free fetal DNA from maternal blood. Clin. Chem. 2011, 57, 1042-1049. [CrossRef] [PubMed]
- Ehrich, M.; Deciu, C.; Zwiefelhofer, T.; Tynan, JA; Cagasan, L.; Tim, R.; Lu, V.; McCullough, R.; McCarthy, E.; Nygren, AO et al. Noninvasive detection of fetal trisomy 21 by sequencing DNA in maternal blood: a study in a clinical setting. US J Obstet Gynecology. 2011, 204, e1-e11. [CrossRef] [PubMed]
- Jensen, TJ;Zwiefelhofer, T.;Tim, RC;Dzakula, Z.;Kim, SK;Mazloom, AR;Zhu, Z.;Tynan, J.;Lu, T.;McLennan, G.;High-throughput parallel sequencing for detection of fetal aneuploidies from maternal plasma. PLoS ONE 2013, 8, e57381. [CrossRef]
- Juneau, K.;Bogard, PE;Huang, S.;Mohseni, M.;Wang, ET;Ryvkin, P.;Kingsley, C.;Struble, CA;Oliphant, A.;Zahn, JM.Microarray-based cell-free DNA analysis improves noninvasive prenatal testing. Fetal Diagnosis. Ther. 2014, 36, 282-286. [CrossRef]
- Zimmermann, B.; Hill, M.; Gemelos, G.; Demko, Z.; Banjevic, M.; Baner, J.; Ryan, A.; Sigurjonsson, S.; Chopra, N.; Dodd, M.; et al. Noninvasive prenatal aneuploidy testing of chromosomes 13, 18, 21, X, and Y using targeted sequencing of polymorphic loci. Prenatal. Diagn. 2012, 32, 1233-1241. [CrossRef]
- Pergament, E., Cuckle, H., Zimmermann, B., Banjevic, M., Sigurjonson, S., Ryan, A., Hall, MP, Dodd, M., Lacroute, P., Stosic, M., et al. Single-nucleotide polymorphism-based noninvasive prescreening in high- and low-risk cohorts. Obstet. Gynecology. 2014, 124, 210-218. [CrossRef]
- Fan, HC; Blumenfeld, YJ; Chitkara, U.; Hudgins, L.; Quake, SR Analysis of size distribution of fetal and maternal cell-free DNA by paired-end sequencing. Clin Chem 2010, 56, 1279-1286. [CrossRef]
- Lo, YM;Chan, KC;Sun, H.;Chen, EZ;Jiang, P.;Lun, FM;Zheng, YW;Leung, TY;Lau, TK;Cantor, CR;et al. Maternal plasma DNA sequencing reveals genome-wide genetic and mutational profiles in the fetus. Sci Trans Med. 2010, 2, 61ra91. [CrossRef]
- Palomaki, GE;Kloza, EM Prenatal cell-free DNA screening test failure: A systematic review of failure rates, risk of Down syndrome, and impact of repeat testing. Genet. Med. 2018, 20, 1312-1323. [CrossRef] [PubMed]
- Jiang, F.; Ren, J.; Chen, F.; Zhou, Y.; Xie, J.; Dan, S.; Su, Y.; Xie, J.; Yin, B.; Su, W.; et al. Noninvasive fetal trisomy (NIFTY) testing: an advanced noninvasive prenatal diagnostic method for fetal autosomal and sex chromosomal aneuploidies. BMC Med. Genome 2012, 5, 57. [CrossRef] [PubMed]
- Liao, C.;Yin, AH;Yin, Peng, CF;Fu, Fu, Yang, JX;Li, R.;Chen, YY;Luo, DH;Zhang, YL;Ou, YM. Noninvasive prenatal diagnosis of common aneuploidies by semiconductor sequencing. Proc Natl Affirm. Sci U S 2014, 111, 7415-7420. [CrossRef] [PubMed]
- Stumm, M.; Entezami, M.; Haug, K.; Blank, C.; Wustemann, M.; Schulze, B.; Raabe-Meyer, G.; Hempel, M.; Schelling, M.; Ostermayer, E.; et al. Diagnostic accuracy of random massively parallel sequencing for noninvasive prenatal detection of common autosomal aneuploidies: a European collaborative study. Prenatal. Diagn. 2014, 34, 185-191. [CrossRef] [PubMed]
- Samango-Sprouse, C.; Banjevic, M.; Ryan, A.; Sigurjonsson, S.; Zimmermann, B.; Hill, M.; Hall, MP; Westemeyer, M.; Saucier, J.; Demko, Z.; et al. A SNP-based noninvasive prenatal test detects sex chromosome aneuploidies with high accuracy. Prenatal. Diagn. 2013, 33, 643-649. [CrossRef]
- Mazloom, A.R.; Dzakula, Z.; Oeth, P.; Wang, H.; Jensen, T.; Tynan, J.; McCullough, R.; Saldivar, J.S.; Ehrich, M.; van den Boom, D.; et al. 母体血漿からの循環無細胞DNAの配列決定による性染色体異数性の非侵襲的出生前検出。 出生前。 Diagn. 2013, 33, 591-597. [CrossRef]
- Helgeson, J.; Wardrop, J.; Boomer, T.; Almasri, E.; Paxton, WB; Saldivar, JS; Dharajiya, N.; Monroe, TJ; Farkas, DH; Grosu, DS; et al. Clinical outcomes of subchronic events detected by genome-wide noninvasive prenatal testing. Prenatal. Diagn. 2015, 35, 999-1004. [CrossRef]
- Martin, K., Iyengar, S., Kalyan, A., Lan, C., Simon, AL, Stosic, M., Kobara, K., Ravi, H., Truong, T., Ryan, A., et al. Clinical experience with single-nucleotide polymorphism-based noninvasive prenatal testing for clinically significant microdeletions. Clin. Genet. 2018, 93, 293-300. [CrossRef]
- van der Meij, KRM; Sistermans, EA; Macville, MVE; Stevens, SJC; Bax, CJ; Bekker, MN; Bilardo, CM; Boon, EMJ; Boter, M.; Diderich, KEM; et al. TRIDENT-2: nationwide implementation of genome-wide noninvasive prenatal testing as a first-line screening test in the Netherlands. US J. Hum. Genet. 2019, 105, 1091-1101. [CrossRef]
- Pertile, MD; Halks-Miller, M.;Flowers, N.; Barbacioru, C.; Kinnings, SL; Vavrek, D.; Seltzer, WK; Bianchi, DW Reare Autosomal trisomies revealed by maternal plasma DNA sequencing suggest increased risk of fetoplacental disease. Transl. Med. 2017, 9. [CrossRef]
- Pescia, G.; Guex , N. ; Iseli, C.; Brennan , L. ; Oysters, M.; Xenarius, I.; Farinelli , L. ; Conrad, B. Cell-free DNA testing of chromosomal anomaly : identification of gene 6388 gene Genet. Med. 2017, 19, 169–175. [CrossRef]
- Bayindir, B.; Dehaspe, L.; Brison, N.; Brady, P.; Ardui, S.; Kammoun, M.; Van der Veken, L.; Lichtenbelt, K.; Van den Bogaert, K.; Van Houdt, J.; Non-invasive prenatal testing using a novel analytical pipeline to screen for all autosomal fetal aneuploidies improves pregnancy management. Eur. J. Hum. Genet. 2015, 23, 1286-1293. [CrossRef] [PubMed]
- Van Opstal, D.; van Maarle, MC; Lichtenbelt, K.; Weiss, MM; Schuring-Blom, H.; Bhola, SL; Ho.er, MJV; Huijsdens-van Amsterdam, K.; Macville, MV; Kooper, AJA; et al. Origin and clinical significance of common non-trisomic chromosomal abnormalities detected by genome-wide NIPS: results from the TRIDENT study. Genet. Med. 2018, 20, 480-485. [CrossRef]
- Scott, F.; Bonifacio, M.; Sandow, R.; Ellis, K.; Smet, M. E.; McLennan, A. Reare Autosomal trisomies: important and not so rare. Prenatal. Diagn. 2018, 38, 765-771. [CrossRef] [PubMed]
- Chatron, N.; Till, M.; Abel, C.; Bardel, C.; Ramond, F.; Sanlaville, D.; Schluth-Bolard, C. Detection of rare autosomal trisomies by noninvasive prenatal testing: Benefits for pregnancy management Ultrasound. Gynecology. 2019, 53, 129-130. [CrossRef] [PubMed]
- Holland, AJ; Cleveland, DW Chromosome formation and cancer: mechanisms and consequences of localized and complex chromosomal rearrangements. Nat. Med. 2012, 18, 1630-1638. [CrossRef] [PubMed]
- Grati, F. R. Chromosomal mosaicism in human fetoplacental development: implications for prenatal diagnosis. J. Clin. Med. 2014, 3, 809-837. [CrossRef]
- Gil, MM; Accurti, V.; Santacruz, B.; Plana, MN; Nicolaides, KH Analysis of cell-free DNA in maternal blood for aneuploidy screening: an updated meta-analysis. Ultrasound. Gynecology. Gynecol. 2017, 50, 302-314. [CrossRef]
- de Wergifosse, S.; Bevilacqua, E.; Mezela, I.; El Haddad, S.; Gounongbe, C.; de Marchin, J.; Maggi, V.; Conotte, S.; Badr, DA; Fils, JF; Analysis of cell-free DNA in maternal blood: genome-wide versus targeted approaches as a primary screening test. J. Matern. Fetal Neonatal Med. 2019. [CrossRef]
- Grati, FR; Ferreira, J.; Benn, P.; Izzi, C.; Verdi, F.; Vercellotti, E.; Dalpiaz, C.; D’Ajello, P.; Filippi, E.; Volpe, N.; et al. Outcome of pregnancies disrupted by placental mosaicism and implications for prenatal screening with cell-free DNA. Genet. Med. 2020, 22, 309-316. [CrossRef]
- Gadsbl, K.; Petersen, O.B.; Gatinois, V.; Strange, H.; Jacobsson, B.; Wapner, R.; Vermeesch, J.R.; Group, T.N.-m.S.; Vogel, I. Current use of noninvasive prenatal testing in Europe, Australia and the USA: A graphical presentation. Acta Obstet. Gynecol. Scand. 2020, 99, 722-730. [CrossRef]
- Benachi, A.;Ca.rey, J.;Carreras, P.; Carreras, E.; Jani, JC; Kilby, MD; Klein, HG; Rizzo, G.; Yaron, Y. Understanding attitudes and behaviors toward cell-free DNA-based noninvasive prenatal testing (NIPT): a survey of European healthcare providers. Eur. J. Med. Genet. 2020, 63, 103616. [CrossRef] [PubMed]
- Ehrich, M.; Tynan, J.; Mazloom, A.; Almasri, E.; McCullough, R.; Boomer, T.; Grosu, D.; Chibuk, J. Genome-wide cfDNA screening: Clinical laboratory experience with the frst 10,000 cases. Genet. Med. 2017, 19, 1332-1337. [CrossRef] [PubMed]
- Liang, D.; Cram, DS; Tan, H.; Linpeng, S.; Liu, Y.; Sun, H.; Zhang, Y.; Tian, F.; Zhu, H.; Xu, M.; et al. Clinical utility of noninvasive prenatal screening for expanded chromosomal disorder syndromes. Genet Median 2019. [CrossRef] [PubMed]
- Xue, Y.; Zhao, G.; Li, H.; Zhang, Q.; Lu, J.; Yu, B.; Wang, T. A noninvasive prenatal test for detecting chromosomal aneuploidies in 57,204 pregnancies. Mol Cytogenetics. 2019, 12, 29. [CrossRef] [PubMed]
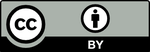
© 2020 by the authors. Licensee MDPI, Basel, Switzerland. This article is an open access article distributed under the terms and conditions of the Creative Commons Attribution (CC BY) license (http://creativecommons.org/licenses/by/4.0/).