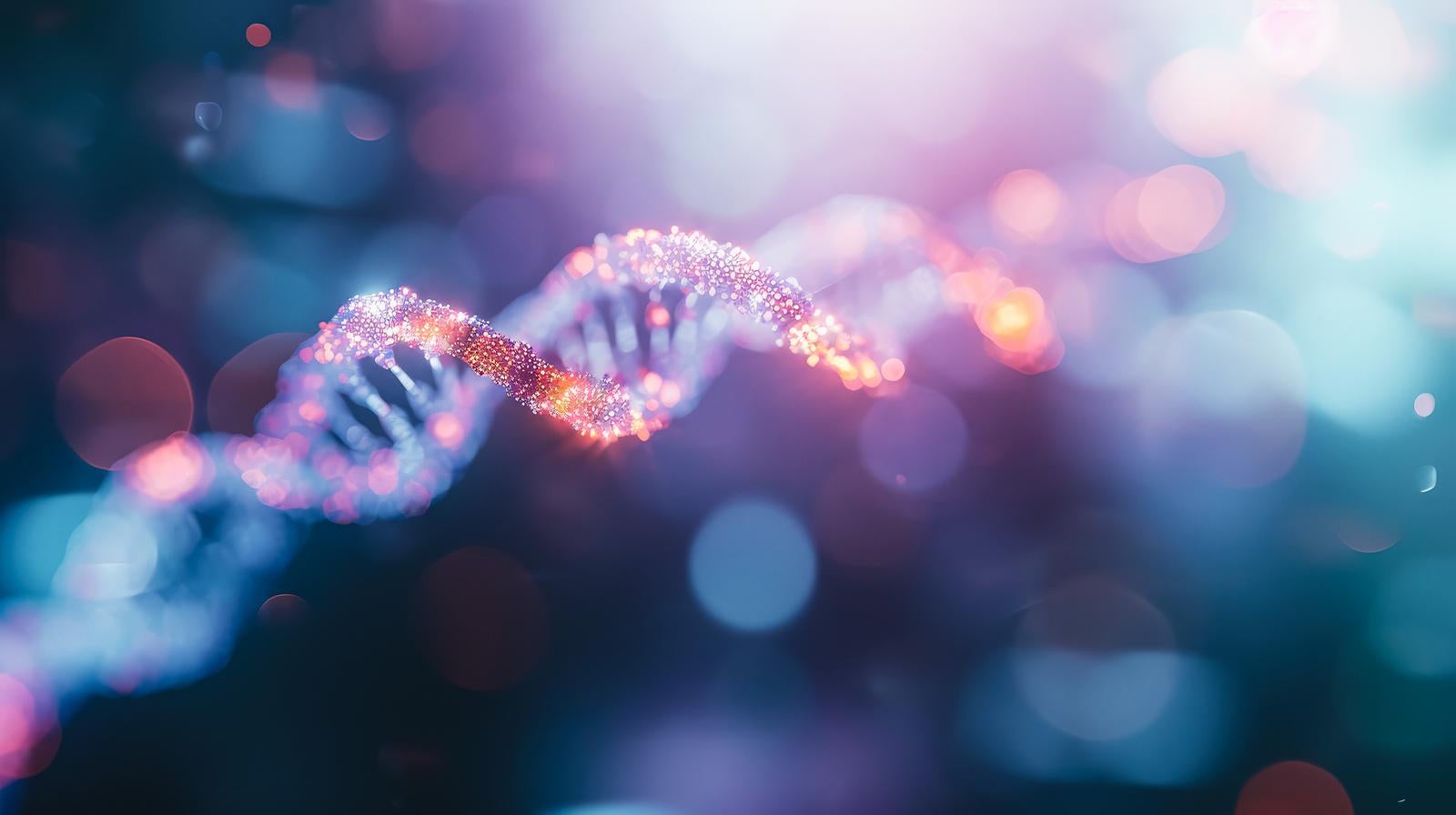
Hiro 诊所的检测精度(父权确认率)的目标是
目标是达到99.99999999999%以上。
这是根据地球上80亿人口来计算的,不同的可能性是0.008人。这个结果是通过统计学中的尤度比得出的,利用STR方法可以实现这个数字。
尤度比与父权确认率的关系
尤度比 | 父权确认率 | 错误概率 |
---|---|---|
10 | 90%以上 | 10人中1人 |
1,000(零三个) | 99.9%以上(九三个) | 1000人中1人 |
100,000(零五个) | 99.999%以上(九五个) | 10万人中1人 |
10,000,000(零七个) | 99.99999%以上(九七个) | 1000万人中1人 |
1,000,000,000(零九个) | 99.9999999%以上(九九个) | 10亿人中1人 |
100,000,000,000(零11个) | 99.999999999%以上(九11个) | 1000亿人中1人 |
10,000,000,000,000(零13个) | 99.99999999999%以上(九13个) | 10万亿人中1人 |
STR因其数值分布具有一定的范围,因此能够更准确地进行鉴别。
要达到这种精度,单独使用SNP需要超过5000个SNP。
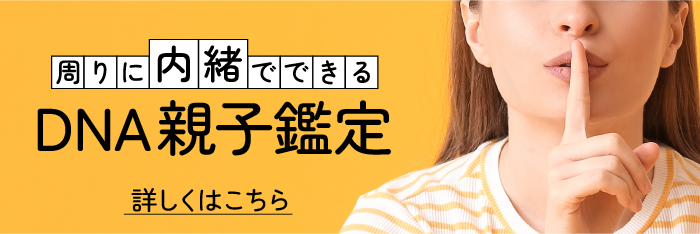
现代DNA亲子鉴定技术的发展
ABO血型系统于1900年被发现,并在1930年代开始用于排除亲子鉴定中的不匹配。随后,Rh血型抗原、MNS血型抗原、人类白血球抗原(HLA)等血液标记物的引入提高了鉴定的准确性,但仍无法完全消除误差。
1977年,采用桑格法进行DNA序列分析的技术出现,DNA检测成为可能,并在亲子鉴定中实现了超越传统方法的统计学准确性。如今,DNA检测已广泛应用于亲子鉴定、法医学调查以及产前检查等多个领域。
检测流程
在诉讼程序中使用检测结果时,相关人员的样本由医生在诊所中采集。其他情况下,可以使用简单易用的试剂盒,在家中自行采集样本并邮寄。
收到样本后,实验室将其录入数据库。随后,采集的细胞内的DNA将被提取和纯化。这一过程通过高通量、完全自动化的系统进行,比传统的DNA检测更快、更经济,且精度更高。
此外,采用针对特定标记基因位点的检测方法,而非对全基因组进行分析,从而在保持精度的同时,大大减少了检测时间和成本。
这些特定标记基因位点被称为“STR(短串联重复序列)”,在扩增后,将用于比较以确认亲属关系(父子关系、母子关系、兄弟关系等)。
什么是STR?
STR是指短串联重复序列(Short Tandem Repeats),它是DNA序列中连续重复的短小相同序列单位。
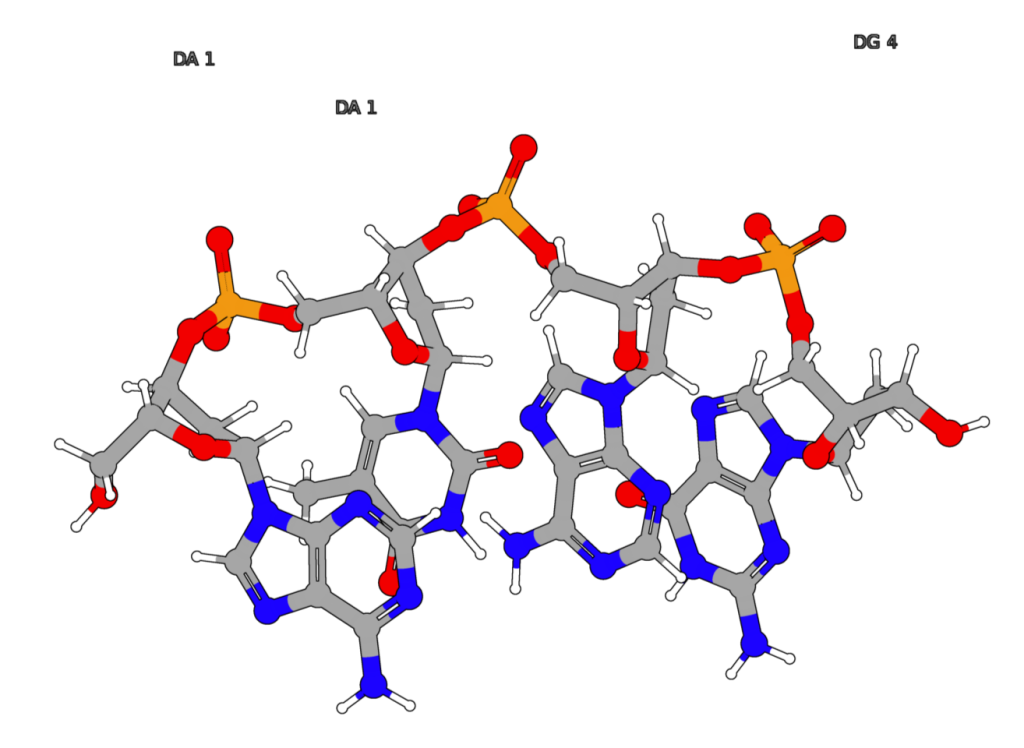
图1:在CSF1PO这个STR中,短序列AGAT重复5到17次。
图2:D3S1358、D4S2408和FGA三个STR的示意图。虽然STR的种类很多,但每个人通常拥有每种STR的两种变体,一个来自父亲,一个来自母亲。
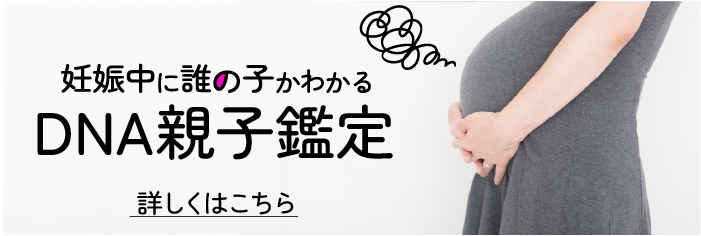
STR分析方法
具有特定多态性的短串联重复序列(STR)基因位点,通过两步聚合酶链式反应(PCR)标记后,进行靶向型下一代测序(NGS)。这种高深度的读取和灵敏度使得即使是使用过的牙刷、吸管等样本,也能成功检测。我们的检测包括了FBI的复合DNA索引系统(CODIS)核心20个STR标记,此外还分析了27个常染色体STR和作为性别判定标记的阿米洛基因(Amelogenin),以及25个Y染色体STR,提供更全面、更精确的结果。
阿米洛基因广泛用于法医学和产前诊断中的性别判定,但通过增加额外的25个Y染色体标记,我们进一步提高了性别判定的准确性和可靠性。
我们采用了为法医学基因组学设计、开发和验证的首个下一代测序(NGS)设备——MiSeq FGx测序系统。该系统经过优化的基因组面板测序完成后,通过集成的Universal Analysis Software(UAS)平台进行数据分析。该平台使用独特的比对算法,检查引物序列(正向和反向)以及STR重复区域的邻接区域。在基因座、等位基因和基因型的确定上,采用的是扩增片段的完整序列,而非片段长度,从而提高了精度。
低于特定阈值的读取计数会被排除,而超过阈值的则被视为等位基因。我们还会考虑群体中的等位基因频率、连锁不平衡以及同一染色体上STR的分布等关键因素,严格分析测序数据。这些步骤确保了获得坚实、灵敏且高精度、可靠的结果。
所使用的系统和试剂盒经过开发验证测试,并符合欧洲标准、SWGDAM(科学工作小组)、CODIS(复合DNA索引系统)、国际刑警(Interpol)和ESS(欧洲标准)的最低要求。
计算方法
人类每条常染色体都有两条,分别从生物学父母那里继承各一条。此外,男性从生物学父亲那里继承Y染色体。无论是生物学母亲还是父亲,胎儿与他们的STR(短串联重复序列)几乎是完全一致的。这个原理如表1所示。
表1. 生物学父母将遗传信息传递给子代。
常染色体STR | Y性染色体 | ||||||||
---|---|---|---|---|---|---|---|---|---|
生物的父亲 | 非生物的父亲 | 生物的父亲 | 非生物的父亲 | ||||||
性别 | D1S1656 | TPOX | D1S1656 | TPOX | DYS393 | DYS505 | DYS393 | DYS505 | |
胎儿 | XY | 10,14 | 10,11 | 10,16 | 10,11 | 14 | 12 | 14 | 12 |
假定的母亲 | XX | 10,14 | 9,10 | 10,14 | 9,10 | ||||
假定的父亲 | XY | 14,14 | 11,11 | 12,12 | 8,8 | 14 | 12 | 13 | 11 |
外遇对象 | XY | – | – | ?, 16 | ?, 11 | – | – | 14 | 12 |
由独特的UAS(通用分析软件)执行的生物信息学分析以及用于亲属关系分析的统计算法超出了本文的范围。对于有兴趣了解数学细节的读者,请参阅以下参考文献,以了解所使用方法的概述。
- Huston, K. A. (1998). Statistical analysis of STR data. Profiles DNA, 1(3), 14-15.
- Evett, Ian, and B. S. Weir. Interpreting DNA Evidence: Statistical Genetics for Forensic Scientists. Sinauer Associates, 1998.
- Gjertson, D. W., Brenner, C. H., Baur, M. P., Carracedo, A., Guidet, F., Luque, J. A., … Morling, N. (2007). ISFG: Recommendations on biostatistics in paternity testing. Forensic Science International: Genetics, 1(3-4), 223–231. doi:10.1016/j.fsigen.2007.06.006
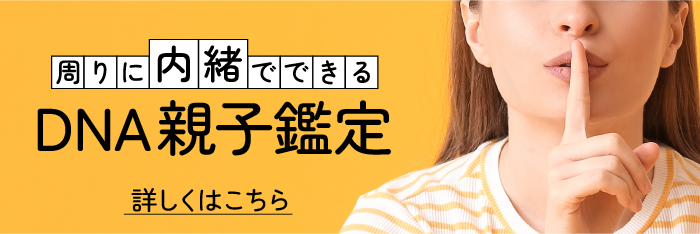
目标STR面板和具体例子
表2. 检测的常染色体STR 27个(包括CODIS核心STR 20个)、Y性染色体STR 25个、以及用于性别判定的阿美洛基因(Amelogenin)标记。
s/n | STR基因座 | 重复序列的重复次数 | 扩增产物的长度(bp) | 染色体 |
---|---|---|---|---|
A1 | D1S1656 | 7–21.3 | 133–192 | 1 |
A2 | TPOX | 4–16 | 61–109 | 2 |
A3 | D2S441 | 7–17 | 137–177 | 2 |
A4 | D2S1338 | 10–33.1 | 110–203 | 3 |
A5 | D3S1358 | 8–22 | 138–194 | 3 |
A6 | D4S2408 | 8–13 | 98–118 | 4 |
A7 | FGA | 12.2–53 | 150–312 | 4 |
A8 | D5S818 | 4–20 | 98–162 | 5 |
A9 | CSF1PO | 5–17 | 72–120 | 5 |
A10 | D6S1043 | 8–26 | 154–226 | 6 |
A11 | D7S8202 | 5–21.1 | 118–183 | 7 |
A12 | D8S1179 | 6–20 | 82–138 | 8 |
A13 | D9S1122 | 8–15 | 104–132 | 9 |
A14 | D10S1248 | 7–20 | 124–176 | 10 |
A15 | TH01 | 3–14 | 96–140 | 11 |
A16 | vWA | 11–26 | 135–195 | 12 |
A17 | D12S391 | 13–28 | 229–289 | 12 |
A18 | D13S317 | 5–17 | 138–186 | 13 |
A19 | PentaE | 5–28.4 | 362–481 | 15 |
A20 | D16S539 | 4–17 | 132–184 | 16 |
A21 | D17S1301 | 9–15 | 130–154 | 17 |
A22 | D18S51 | 6–40 | 136–272 | 18 |
A23 | D19S433 | 4–27 | 148–240 | 19 |
A24 | D20S482 | 9–17 | 125–157 | 20 |
A25 | D21S11 | 12–41.2 | 147–265 | 21 |
A26 | PentaD | 1.1–19 | 209–298 | 21 |
A27 | D22S1045 | 8–19 | 201–245 | 22 |
Y1 | DYF387S1 | 30–44 | 207–263 | Y |
Y2 | DYS19 | 9–19 | 269–309 | Y |
Y3 | DYS385a-b | 7–28 | 232–316 | Y |
Y4 | DYS389I | 9–17 | 236–268 | Y |
Y5 | DYS389II | 24–34 | 283–323 | Y |
Y6 | DYS390 | 17–28 | 290–334 | Y |
Y7 | DYS391 | 5–16 | 119–163 | Y |
Y8 | DYS392 | 6–17 | 318–362 | Y |
Y9 | DYS393 | 9–18 | 108–144 | Y |
Y10 | DYS437 | 10–18 | 194–226 | Y |
Y11 | DYS438 | 6–16 | 129–179 | Y |
Y12 | DYS439 | 6–17 | 167–211 | Y |
Y13 | DYS448 | 14–26 | 330–402 | Y |
Y14 | DYS460 | 7–14 | 348–376 | Y |
Y15 | DYS481 | 17–32 | 129–174 | Y |
Y16 | DYS505 | 9–15 | 162–186 | Y |
Y17 | DYS522 | 8–17 | 298–334 | Y |
Y18 | DYS533 | 7–17 | 186–226 | Y |
Y19 | DYS549 | 10–14 | 210–226 | Y |
Y20 | DYS570 | 10–26 | 142–206 | Y |
Y21 | DYS576 | 10–25 | 163–223 | Y |
Y22 | DYS612 | 26–33 | 275–296 | Y |
Y23 | DYS635 | 15–30 | 242–302 | Y |
Y24 | DYS643 | 7–15 | 141–181 | Y |
Y25 | Y-GATA-H4 | 8–15 | 159–187 | Y |
性别决定 | 钙质蛋白 | – | – | X/Y |
表3. 虚拟例子1&2(假设的非生物学父亲/假设的生物学父亲)
假设案例 1: 假定的非生物学父亲 | 性别 | D1S1656 | TPOX | D2S441 | D2S1338 | D3S1358 | D4S2408 | FGA | D5S818 | CSF1PO | D6S1043 | D7S820 | D8S1179 | D9S1122 | D10S1248 | TH01 | vWA | D12S391 | D13S317 | PentaE | D16S539 | D17S1301 | D18S51 | D19S433 | D20S482 | D21S11 | PentaD | D22S1045 | Amelogenin | |
常染色体 STR+铸牙蛋白 | 胎儿 | XY | 10,14 | 10,11 | 11,14 | 21,22 | 17,18 | 10,10 | 23,23 | 12,13 | 12,12 | 17,23 | 10,10 | 14,14 | 11,12 | 12,16 | 7,8 | 15,19 | 18,21 | 12,12 | 8,9 | 11,12 | 12,12 | 15,17 | 13,13 | 14,15 | 28,28 | 5,8 | 17,17 | X,Y |
假定的母亲 | XX | 10,14 | 9,10 | 11,14 | 20,21 | 17,18 | 10,10 | 23,24 | 12,14 | 11,12 | 16,23 | 9,10 | 14,14 | 11,12 | 12,16 | 6,8 | 14,19 | 18,21 | 11,12 | 7,9 | 10,12 | 12,12 | 15,18 | 12,13 | 13,15 | 27,28 | 5,7 | 17,18 | X,X | |
假定的非生物学父亲 | XY | 12,12 | 8,8 | 12,13 | 19,20 | 16,16 | 9,11 | 22,22 | 11,12 | 11,13 | 15,16 | 11,12 | 13,15 | 10,13 | 14,17 | 6,9 | 17,18 | 16,17 | 9,11 | 10,12 | 9,10 | 10,11 | 12,14 | 12,14 | 13,14 | 29,30 | 7,9 | 16,18 | X,Y | |
性别 | DYS393 | DYS505 | DYS570 | DYS576 | DYS522 | DYS481 | DYF387S1 | DYS19 | DYS391 | DYS635 | DYS437 | DYS439 | DYS389II | DYS389I | DYS438 | DYS612 | DYS390 | DYS643 | DYS533 | Y-GATA-H4 | DYS385a-b | DYS460 | DYS549 | DYS392 | DYS448 | |||||
Y 性染色体 | 胎儿 | XY | 14 | 12 | 16 | 18 | 11 | 25 | 38,39 | 16 | 10 | 21 | 13 | 13 | 31 | 13 | 11 | 36 | 21 | 13 | 11 | 11 | 17,18 | 11 | 11 | 11 | 29 | 31 | ||
假定的母亲 | XX | 11 | 10 | 11 | 11 | 9 | 18 | 31 | 13 | 13 | 15 | 16 | 12 | 14 | 18 | 16 | 13 | 11 | 11 | 11 | 15 | 17 | 12 | 13 | 13 | 29 | 32 | |||
假定的非生物学父亲 | XY | 17 | 10 | 11 | 16 | 11 | 15 | 11 | 11 | 12 | 11 | 14 | 17 | 15 | 13 | 12 | 9 | 16 | 17 | 20 | 11 | 17 | 13 | 16 | 14 | 11 | 28 | 34 |
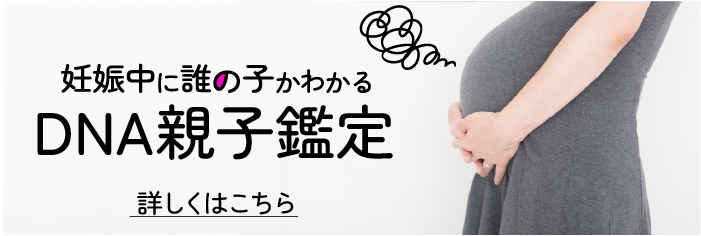
参考资料
- Kane K. (1982). Paternity exclusion and probability of paternity. Annals of clinical and laboratory science, 12(4), 309–314.
- Bugert, P., Rink, G., Kemp, K., & Klüter, H. (2012). Blood Group ABO Genotyping in Paternity Testing. Transfusion medicine and hemotherapy : offizielles Organ der Deutschen Gesellschaft fur Transfusionsmedizin und Immunhamatologie, 39(3), 182–186. https://doi.org/10.1159/000339235
- S. Arnott, P.J. Campbell-Smith & R. Chandrasekaran. Handbook of Biochemistry and Molecular Biology, 3rd ed. Nucleic Acids–Volume II, G.P. Fasman, Ed. Cleveland: CRC Press, (1976). pp. 411-422.
- Schrödinger, L., & DeLano, W. (2020). The PyMOL Molecular Graphics System, Version 3.1.0a0. Schrödinger, LLC.. Retrieved from http://www.pymol.org/pymol
- Tomasello, Gianluca, et al. ‘The Protein Imager: A Full-Featured Online Molecular Viewer Interface with Server-Side HQ-Rendering Capabilities’. Bioinformatics, edited by Arne Elofsson, vol. 36, no. 9, May 2020, pp. 2909–11. DOI.org (Crossref), https://doi.org/10.1093/bioinformatics/btaa009.
- Stephens, Kathryn M., et al. ‘Developmental Validation of the ForenSeq MainstAY Kit, MiSeq FGx Sequencing System and ForenSeq Universal Analysis Software’. Forensic Science International: Genetics, vol. 64, May 2023, p. 102851. DOI.org (Crossref), https://doi.org/10.1016/j.fsigen.2023.102851.
- Thangaraj, K., Reddy, A. G., & Singh, L. (2002). Is the amelogenin gene reliable for gender identification in forensic casework and prenatal diagnosis?. International journal of legal medicine, 116(2), 121–123. https://doi.org/10.1007/s00414-001-0262-y
- Jäger, AC, et al. Developmental validation of the MiSeq FGx Forensic Genomics System for targeted next generation sequencing in forensic DNA casework and database laboratories. Forensic Sci Int Genet. 2017; 28: 52–70. doi.org/10.1016/j.fsigen.2017.01.011.
- Nakazato T, Ohta T, Bono H. Experimental design-based functional mining and characterization of high-throughput sequencing data in the sequence read archive. PLoS One. 2013; 8 (10): e77910. doi.org/10.1371/journal.pone.0077910.
- Ballard D, Winkler-Galicki J, Wesoły J. Massive parallel sequencing in forensics: advantages, issues, technicalities, and prospects. Int J Legal Med. 2020; 134: 1291–1303.doi.org/10.1007/s00414-020-02294-0.
- Ingold S, et al. Body fluid identification using a targeted mRNA massively parallel sequencing approach – results of a EUROFORGEN/EDNAP collaborative exercise. Forensic Sci Int Genet. 2018; 34: 105–115. doi.org/10.1016/j.fsigen.2018.01.002.
- Bentley, DR, et al. Accurate whole human genome sequencing using reversible terminator chemistry. Nature. 2008; 456: 53–59. doi.org/10.1038/nature07517.
- Huston, K. A. (1998). Statistical analysis of STR data. Profiles DNA, 1(3), 14-15.
- Evett, Ian, and B. S. Weir. Interpreting DNA Evidence: Statistical Genetics for Forensic Scientists. Sinauer Associates, 1998.
- Gjertson, D. W., Brenner, C. H., Baur, M. P., Carracedo, A., Guidet, F., Luque, J. A., … Morling, N. (2007). ISFG: Recommendations on biostatistics in paternity testing. Forensic Science International: Genetics, 1(3-4), 223–231. doi:10.1016/j.fsigen.2007.06.006